Table of Contents
Quantum computing has emerged as a groundbreaking field that holds immense potential to revolutionize the way we solve complex problems. With its ability to harness the power of quantum mechanics, this technology has the potential to outperform classical computers in various domains.
Understanding Quantum Computing
Traditional computers, known as classical computers, rely on bits to store and process information. A bit can represent either a 0 or a 1. In contrast, quantum computers leverage quantum bits, or qubits, which can exist in multiple states simultaneously thanks to a phenomenon known as superposition. This unique characteristic allows quantum computers to perform vast calculations simultaneously, leading to exponentially faster processing speeds.
The Principles of Quantum Mechanics
To grasp the workings of quantum computing, it’s essential to comprehend some key principles of quantum mechanics. Superposition refers to the ability of qubits to exist in multiple states simultaneously. The concept of entanglement, on the other hand, involves the correlation between multiple qubits, such that their states become interdependent. These principles form the foundation for quantum computing’s remarkable computational power.
Quantum Gates
Analogous to classical computers’ logic gates, quantum computers employ quantum gates to manipulate qubits and perform operations on quantum data. These gates include the Hadamard gate, CNOT gate, Pauli gates, and many others. By applying different gate combinations, quantum computers can perform complex calculations efficiently.
Quantum Algorithms
Quantum algorithms are the building blocks that enable quantum computers to solve specific problems efficiently. One of the most well-known algorithms is Shor’s algorithm, which demonstrates the ability of quantum computers to factor large numbers exponentially faster than classical computers. Other prominent algorithms include Grover’s algorithm for search problems and the Quantum Fourier Transform.
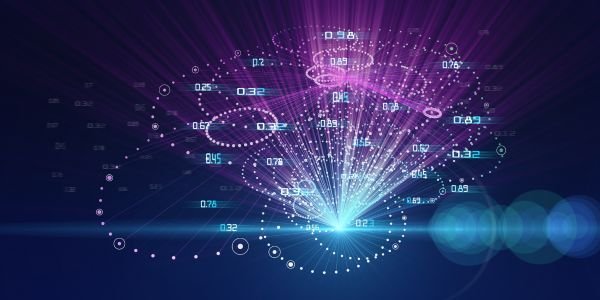
Quantum Computing Implementations
Several physical systems serve as potential platforms for building quantum computers. Superconducting qubits, trapped ion qubits, topological qubits, and photon-based qubits are among the most promising approaches. Each of these systems has unique advantages and challenges, and researchers continue to explore and refine them.
Challenges and Limitations
Despite its immense potential, quantum computing faces numerous challenges and limitations. One of the primary challenges is the issue of decoherence, which arises from environmental interactions and hampers the stability of qubits.
Error correction techniques and fault-tolerant designs are being developed to address this challenge. Additionally, scaling quantum computers to a larger number of qubits while maintaining coherence poses significant technical hurdles.
Applications Quantum computing
1. Quantum Cryptography and Security:
One of the most promising applications of quantum computing lies in cryptography and security. Quantum computers have the potential to break existing cryptographic algorithms, making traditional encryption vulnerable. However, quantum cryptography offers a solution by leveraging the principles of quantum mechanics to establish secure communication channels.
Quantum key distribution (QKD) protocols ensure that encrypted messages remain confidential and tamper-proof, safeguarding sensitive information in the digital age.
2. Optimization and Logistics:
Quantum computing has the potential to revolutionize optimization problems, which are pervasive across various industries. From supply chain management and transportation logistics to financial portfolio optimization, quantum algorithms can provide more efficient solutions.
Quantum annealing, a technique used to find the global minimum of a mathematical function, holds particular promise for tackling optimization challenges. By harnessing the power of quantum computing, businesses can streamline operations, reduce costs, and improve overall efficiency.
3. Drug Discovery and Material Science:
Quantum computing can significantly accelerate the process of drug discovery and development. Simulating the behavior of molecules and predicting their properties accurately is a computationally intensive task. Quantum computers can simulate the quantum behavior of molecules, enabling researchers to model complex molecular interactions and identify potential drug candidates more efficiently.
Moreover, quantum computers can aid in material science research by designing new materials with specific properties, such as high conductivity or superior strength.
4. Machine Learning and Data Analysis:
Quantum computing has the potential to enhance machine learning algorithms and data analysis techniques. Quantum machine learning algorithms can handle vast amounts of data and perform complex computations faster than their classical counterparts.
Quantum algorithms, such as the Quantum Support Vector Machine and Quantum Principal Component Analysis, have shown promising results in tackling complex classification and dimensionality reduction problems. Quantum-enhanced data analysis can unlock valuable insights and drive innovation across industries.
5. Financial Modeling and Risk Analysis:
In the realm of finance, quantum computing can revolutionize risk analysis and financial modeling. The complex nature of financial markets requires advanced computational power to optimize investment portfolios, simulate market scenarios, and assess risk. Quantum algorithms can process large-scale financial data, providing more accurate risk assessments and enabling faster portfolio optimization.
This capability can lead to more informed investment decisions and improved risk management strategies.
6. Quantum Simulations:
Quantum computers excel at simulating quantum systems, which is vital for understanding and predicting the behavior of atoms, molecules, and other quantum systems. Simulating quantum systems using classical computers becomes exponentially challenging as the system size increases.
Quantum simulators can overcome these limitations and shed light on fundamental quantum phenomena, enabling advancements in physics, chemistry, and other scientific disciplines.
What are the 5 main components of quantum computing?
1. Qubits – The Quantum Bits:
At the heart of quantum computing are qubits, the quantum analog of classical bits. Qubits represent the fundamental units of information in a quantum computer. Unlike classical bits, which can only exist as either a 0 or a 1, qubits leverage the principles of quantum mechanics to exist in superposition.
This means that a qubit can be in multiple states simultaneously, enabling quantum computers to process vast amounts of information in parallel. Common physical systems used to implement qubits include superconducting circuits, trapped ions, and topological systems.
2. Quantum Gates – Manipulating Qubits:
Quantum gates play a vital role in manipulating and transforming qubits. Similar to classical logic gates, quantum gates are the building blocks of quantum circuits. They allow for the transformation of qubit states, enabling operations such as superposition, entanglement, and measurements.
Popular quantum gates include the Hadamard gate, which creates superposition, and the CNOT (Controlled-NOT) gate, which entangles two qubits. These gates, along with many others, enable the execution of quantum algorithms and computations.
3. Quantum Registers – Managing Qubits:
Quantum registers are collections of qubits used to store and process quantum information collectively. They act as the quantum equivalent of classical computer registers. Quantum registers provide the necessary framework for executing quantum algorithms by organizing and managing the qubits involved in the computation. They enable simultaneous operations on multiple qubits, allowing for parallel processing and enhancing computational efficiency.
4. Quantum Memory – Preserving Quantum Information:
Quantum memory refers to the ability to store and retrieve quantum information reliably. Maintaining the fragile quantum states of qubits is crucial for the correct execution of quantum algorithms. Quantum memory technologies aim to minimize errors and preserve the coherence of qubits for extended periods. Techniques such as error correction codes and fault-tolerant designs are employed to mitigate the effects of noise, decoherence, and other factors that degrade the integrity of quantum information.
5. Quantum Measurement – Extracting Information:
Quantum measurement is the process of extracting information from qubits. It allows us to obtain classical information by collapsing the superposition of qubit states into classical states. Quantum measurement is an irreversible operation and introduces randomness into the computation.
The measurement outcomes provide the results of quantum computations and are crucial for obtaining meaningful outputs.
Also, read 3D Printing
Comment on “Exploring the Fascinating World of Quantum Computing”
Comments are closed.